Multiscale
modeling of hematological disorders
Hematological
disorders arising from infectious diseases or genetic
factors could lead to significant alterations in the
shape and deformability of circulating red blood cells
(RBCs) as well as rheology of blood flow. Examples of
blood related disorders include sickle cell anemia,
malaria, and hereditary spherocytosis and elliptocytosis.
In silico modeling of hematological disorders
provide a promising means for tackling a broad range
of mechanical and rheological blood related problems.
Examples include dynamic deformability for various stages
of malaria-infected RBCs, and cell morphological sickling
and vaso-occlusion phenomena in sickle cell anemia.
[Movie] |
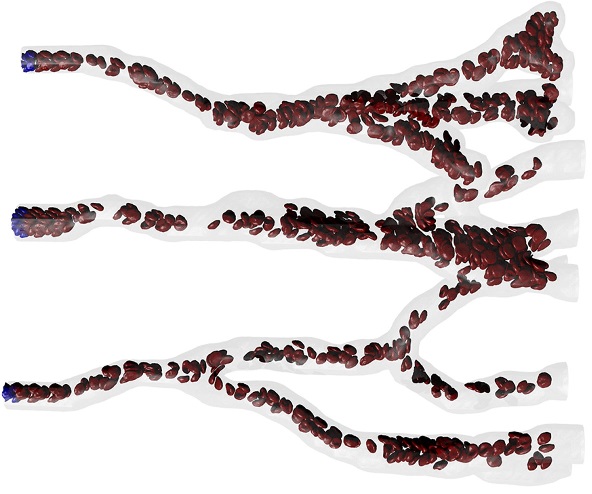 |
Related
publications:
- X. J. Li, P.
M. Vlahovska and G. E. Karniadakis. Continuum- and particle-based
modeling of shapes and dynamics of red blood cells in health
and disease. Soft Matter 2013, 9, 28-37.
- K. Lykov, X.
J. Li, I. V. Pivkin, and G. E. Karniadakis. Inflow/Outflow
boundary conditions for particle-based blood flow simulations:
Application to arterial bifurcations and trees. PLOS
Comput. Biol. 2015, 11, e1004410.
- H. Y. Chang,
X. J. Li, H. Li, and G. E. Karniadakis. MD/DPD multiscale
framework for predicting morphology and stresses of red blood
cells in health and disease. PLOS Comput. Biol. 2016,
12, e1005173.
- X.
J. Li, H. Li, H.-Y. Chang, G. Lykotrafitis, and G. E. Karniadakis.
Computational biomechanics of human red blood cells in hematological
disorders. J. Biomech. Eng. 2017, 139, 020804.
|
Biomechanics
and biorheology of human red blood cells
Human
RBCs have remarkable deformability, squeezing through
narrow capillaries as small as three microns in diameter
without any damage. However, this feature of RBCs can
be critically affected in pathological conditions. Quantification
of the biomechanical and biorheological characteristics
of RBCs can improve our understanding of the etiology
of a number of human diseases. Computational methods
have been used to investigate a broad range of biomechanical
and rheological problems associated with RBCs, to answer
questions concerning the coupling of biochemistry and
mechanics, and the mechanics of diseased RBCs.
[Movie] |
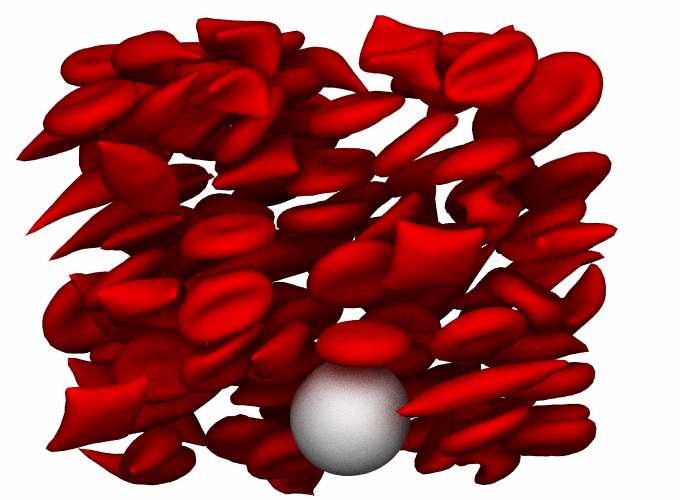 |
Related
publications:
-
Z.
L. Peng, X. J. Li, I. V. Pivkin, M. Dao, G. E. Karniadakis,
and S. Suresh. Lipid-bilayer and cytoskeletal interactions
in a red blood cell. Proc. Natl. Acad. Sci. U.S.A.
2013, 110, 13356-13361.
- X. J. Li, Z. L. Peng, H. Lei, M. Dao, and G. E. Karniadakis.
Probing red blood cell mechanics, rheology and dynamics
with a two-component multiscale model. Philos. T. R.
Soc. A. 2014, 372, 20130389.
- X. J. Li, E. Du, H. Lei, Y.-H. Tang, M. Dao, S. Suresh,
and G. E. Karniadakis. Patient-specific modeling and predicting
blood viscosity in sickle-cell anemia. Interface Focus
2016, 6, 20150065.
-
X.
J. Li, M. Dao, G. Lykotrafitis, and G. E. Karniadakis. Biomechanics
and biorheology of red blood cells in sickle cell anemia.
J. Biomech. 2017, 50, 34-41.
|
Microfluidics
RBC
deformability has been recognized as a sensitive biomarker
for rheological disease. Informed by recent experiments
involving microfluidics that provide in vitro quantitative
information on cell dynamics under transient hypoxia conditions,
we have developed a high-throughput microfluidics-based
model and performed detailed computational simulations of
alterations to cell behavior in response to morphological
changes and membrane stiffening. |
|
Related
publications:
-
X.
J. Li, A. S. Popel, and G. E. Karniadakis. Blood-plasma
separation in Y-shaped bifurcating microfluidic channels: A
dissipative particle dynamics simulation study. Phys. Biol.
2012, 9, 026010.
-
X.
J. Li, E. Du, M. Dao, S. Suresh, and G. E. Karniadakis. Patient-specific
modeling of individual sickle cell behavior under transient
hypoxia. PLOS Comput. Biol. 2017, 13, e1005426.
- A. Blumers, Y.-H. Tang, Z. Li, X. J. Li, and G. E. Karniadakis.
GPU-accelerated red blood cells simulations with transport
dissipative particle dynamics. Comput. Phys. Commun. 2017,
217, 171-179.
-
H.-Y. Chang, X. J. Li, and G. E. Karniadakis.
Modeling of biomechanics and biorheology of red blood
cells in type-2 diabetes mellitus. Biophys. J. 2017,
113, 481-490.
|
Self-assembly
of amphiphiles (polymer/lipid/hemoglobin molecules)
Self-assembly,
which makes use of molecular rather than atomic units,
offers a bottom-up approach to the development of complex
materials at different length scales. The ability to self-assemble
is inherent in biological macromolecules. Chiral molecular
self-assembled structures have been associated with a
number of human diseases, including sickle cell anemia
induced by the growth of polymer fibers and gallstones
formation in nucleating bile. We have developed different
particle-based models to investigate the formation dynamics
of these self-assembled microstructrues.
[Movie] |
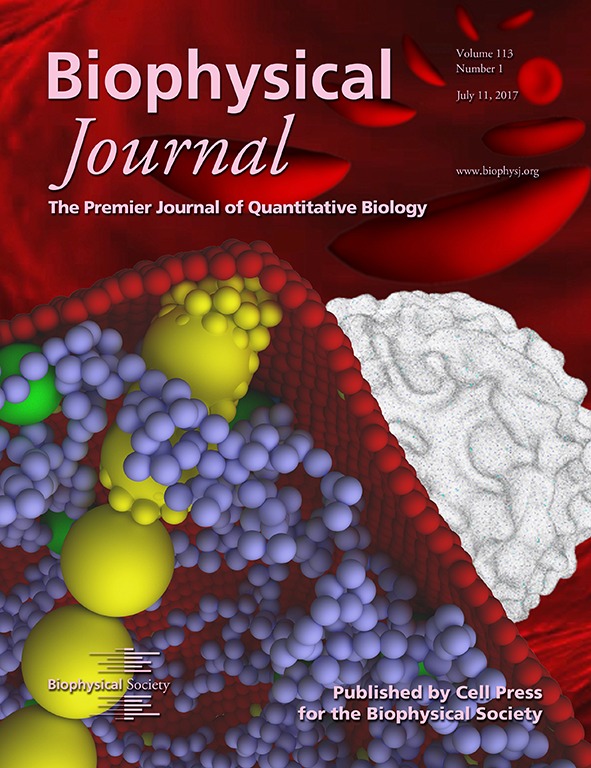 |
Related
publications:
-
X.
J. Li, I. V. Pivkin, H. J. Liang, and G. E. Karniadakis. Shape
transformations of membrane vesicles from amphiphilic triblock
copolymers: A dissipative particle dynamics simulation study.
Macromolecules 2009, 42, 3195-3200.
-
X.
J. Li, B. Caswell, and G. E. Karniadakis. Effect of chain
chirality on the self-assembly of sickle hemoglobin. Biophys.
J. 2012, 103, 1130-1140.
-
X.
J. Li. Shape transformations of bilayer vesicles from amphiphilic
block copolymers: A dissipative particle dynamics simulation
study. Soft Matter 2013, 9, 11663-11670.
-
X.
J. Li, Y.-H. Tang, H. J. Liang, and G. E. Karniadakis. Large-scale
dissipative particle dynamics simulations of self-assembled
amphiphilic systems. Chem. Commun. 2014, 50, 8306-8308.
-
Y.-H. Tang, Z. Li, X. J. Li, M. G. Deng,
and G. E. Karniadakis. Non-equilibrium dynamics of vesicles
and micelles by self-assembly of block copolymers with double
thermoresponsivity. Macromolecules 2016, 49, 2895-2903.
-
L. Lu, H. Li, X. Bian, X. J. Li, and G.
E. Karniadakis. Mesoscopic adaptive resolution scheme toward understanding of interactions between
sickle cell fibers. Biophys. J. 2017, 113, 48-59 (Cover Article).
|
Dynamics
of Polymer/DNA Chain translocation through a microchannel
The
motion of polymer molecules along channels is essential
for many physical and biological processes, recent experimental
advances that allowed to investigate the translocation
of polymers with a single-molecule precision; however,
the understanding of fundamental processes underlying
the translocation phenomena is still quite limited. We
investigate the process of polymer/DNA chain translocation
through a microchannel. |
|
Related
publications:
-
J.
Y. Guo, X. J. Li, Y. Liu, and H. J. Liang. Flow-induced
translocation of polymers through a fluidic channel: A dissipative
particle dynamics simulation study. J. Chem. Phys. 2011,
134, 134906.
-
X.
J. Li, X. L. Li, M. G. Deng, and H. J. Liang. Effects
of electrostatic interactions on the polymer translocation
through a narrow pore under different solvent conditions:
A dissipative particle dynamics simulation study. Macromol.
Theory Sim. 2012, 21, 120-129.
-
X.
J. Li, I. V. Pivkin, and H. J. Liang. Hydrodynamic effects
on flow-induced polymer translocation through a microfluidic
channel. Polymer 2013, 54, 4309-4317.
|
 |
Updated
June 3, 2017 |
|